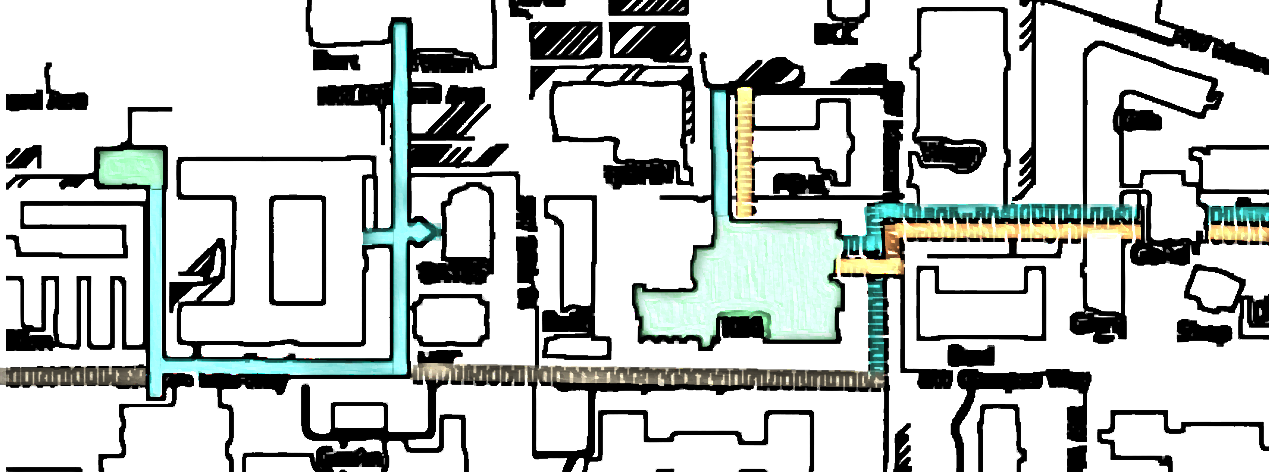
- Published in the International Journal of Energy Management
CASE STUDY: Low Carbon Campus Heating
This case study is based on an article originally written by Systems West and published in the International Journal of Energy Management. We gratefully acknowledge the Association of Energy Engineers (AEE) for granting permission to use and adapt content from the original publication. For further details and to access the full article, please refer to The 2024 International Journal of Energy Management Vol 6 Issue 4.
Learn more about the Association of Energy Engineers by visiting www.aeecenter.org
Learning Objectives
After reading this case study, you should be able to:
- Understand the principles of demand-side management as a
pathway to decarbonization for district-level energy systems. - Estimate the scale of energy savings of a district-level utility
network compared to a traditional campus system. - Consider alternate or supplemental options to decarbonize
central heating plants. - Ensure long-term savings persistence with strong system recommendations.
Project Description
Oregon State University (OSU) in Corvallis, OR has a centralized natural gas-powered Combined Heat and Power (CHP) Energy Center that supplies the campus with steam while generating approximately 40% of the campus’s electricity. With a commitment to reduce greenhouse gas emissions, OSU aims to understand strategies for long-term heating infrastructure, emphasizing the importance of low-carbon heating systems in achieving campus-wide sustainability goals.
Due to the electric production capabilities of OSU’s Energy Center, the University is focusing on demand-side strategies to manage its long-term decarbonization goals. A significant opportunity to advance these goals lies in expanding the scope of their planned chilled-water upgrades. The University is transitioning toward centralizing chilled-water production through strategically placed district utility plants (DUPs) across the campus. This study explores the potential of integrating district hot water systems into these DUPs to create integrated district-level utility networks. By implementing this strategy, centralized heat recovery at the plants can be achieved, significantly reducing reliance on campus steam.
Technology Highlights
An often-underutilized heat source is the return water in district chilled-water systems. The planned DUPs at OSU are primarily intended to operate with traditional central chilled-water systems, where heat in the chilled-water return is rejected to outside air via cooling towers. However, by integrating heat recovery chillers (HRCs) at the planned DUPs, the heat in the chilled-water return can alternatively be used to provide heat to a low-temperature district heating water system. Low-temperature heating water distribution is more compatible with HRCs than high-temperature systems.
Figure 1 below depicts how HRCs integrate with district heating- and chilled-water systems to recover heat.
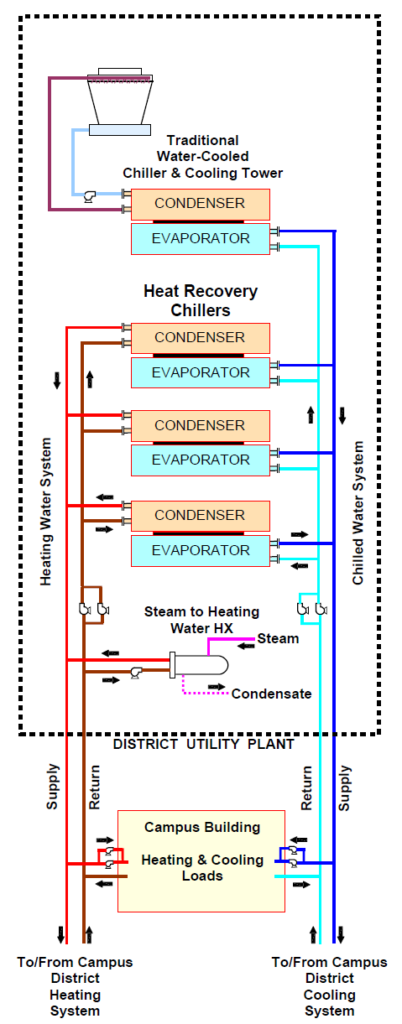
Figure 1 – District-Level Utility Network Conceptual Schematic
Maximizing the heat provided by the HRCs simultaneously minimizes the dependency on gas-fueled steam. The primary strategy, therefore, involves increasing the temperature of the chilled-water return when there is a heating demand on campus. Identifying cooling loads that coincide with heating loads is crucial for this approach. By synchronizing these loads, we can enhance the performance of HRC operation, thus playing a key role in the campus’s decarbonization efforts.
Our Approach
Heating Load Analysis
To properly analyze the amount of annual heating that the district-level utility network can potentially offset from the campus steam system, understanding the classification of campus heating loads was essential. The campus’s steam loads were broken down into three tiers, which helped identify the feasibility of different electrification options and the potential for emissions reduction.
Category 1: Loads compatible with low-quality heating sources such as low-temperature heating water distribution (approximately 1300F or less).
Category 2: Loads requiring high-quality heating sources such as direct steam.
Category 3: Loads associated with the steam systems’ distribution losses. Successful electrification of Category 1 and 2 loads could lead to the elimination of Category 3 loads.
Figure 2 below illustrates the portion of each category that encompasses the annual heating load for OSU. The curve in the figure represents the load duration over an average year at the Corvallis campus. Any point on the load duration curve indicates the number of hours in a typical year that the heating load is at or above a certain demand (in MMBtu/hr of steam in this case).
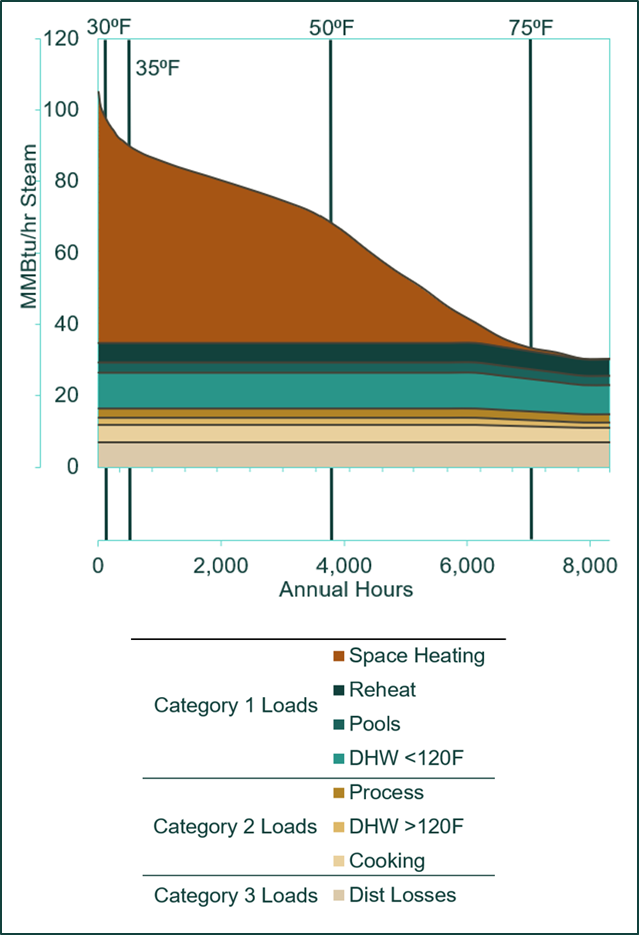
Figure 2 – Campus Heating Load Duration Curve by End Use
The vertical lines in the graph represent the number of hours in a typical year that Corvallis experiences outside air dry bulb temperatures at or below a certain value in degrees Fahrenheit. This graph shows that the peak campus heating demand of approximately 100-110 MMBtu/hr only occurs for a handful of hours each year (below outside air temperatures of 300F). Since the area under the load duration curve represents the total annual heating energy for the OSU Corvallis campus, the curve also shows us that 95% of the heating energy of the campus occurs at outside air dry bulb temperatures above 350F.
Load Reduction Strategies
Category 1 loads represent 79% of the total campus heating energy in steam. With a low-temperature hot water system integrated in the district-level utility networks at the campus DUPs, this energy can be offset using the HRCs by implementing a number of heat recovery options to maximize simultaneous heating and cooling loads:
- Building cooling heat recovery: Heat is rejected to the district cooling system from buildings or zones that have space cooling loads. This heat can be utilized by other buildings and zones that simultaneously require heat.
- Economizer override in buildings: Overriding the economizing operation of buildings will increase the heat that gets rejected to the chilled-water return and ultimately is recovered to buildings and zones requiring heat.
- Lab exhaust heat recovery: Many types of research labs require high air changes per hour (ACH) for exhaust systems to maintain health and safety standards. By implementing chilled-water coils in the laboratory exhaust streams, the campus can capture additional waste heat in the district chilled-water return system instead of expelling it to the outdoors.
- Utility space cooling: Adding chilled-water fan coils to unconditioned spaces on campus that tend to have high ambient temperature is another strategy to increase the loading of heat recovery chillers. In the case of OSU, the underground steam tunnels meet these qualifications.
Figure 3 below is another representation of the campus steam profile. Both campus steam demand (in MMBtu/hr) and annual hours are plotted against outside air dry bulb temperatures.
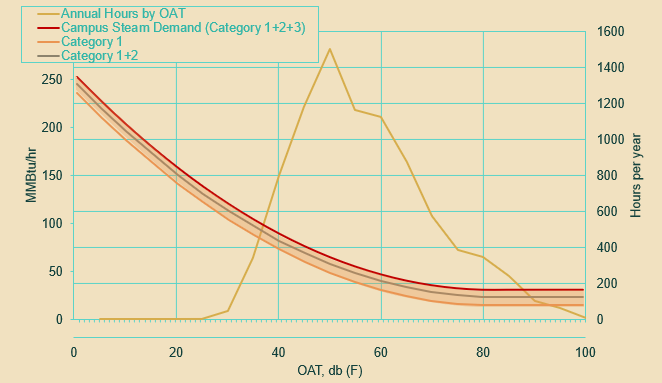
Figure 3 – Campus Heating Load Versus Outside Air Temperature
Figure 4 illustrates a feasible long-term concept that helps quantify the portion of the campus heating load that can effectively be served via the heat recovery options described above along with supplemental concepts.
The goal of the integrated concept is to combine strategies to meet all campus Category 1 heating loads above approximately 300F outside air dry bulb temperatures. These loads account for 79% of the campus heating energy in a typical year. Each strategy is represented in a solid color to the left of the heating load curve and hatched to the right of the curve. The hatched area represents the non-coincident heating potential for each strategy. For example, there is plenty of waste heat due to building space cooling when the outdoor air dry bulb temperature is above 900F, but the heating requirement of the campus is much lower, so the hatched blue area is unrecoverable waste heat.
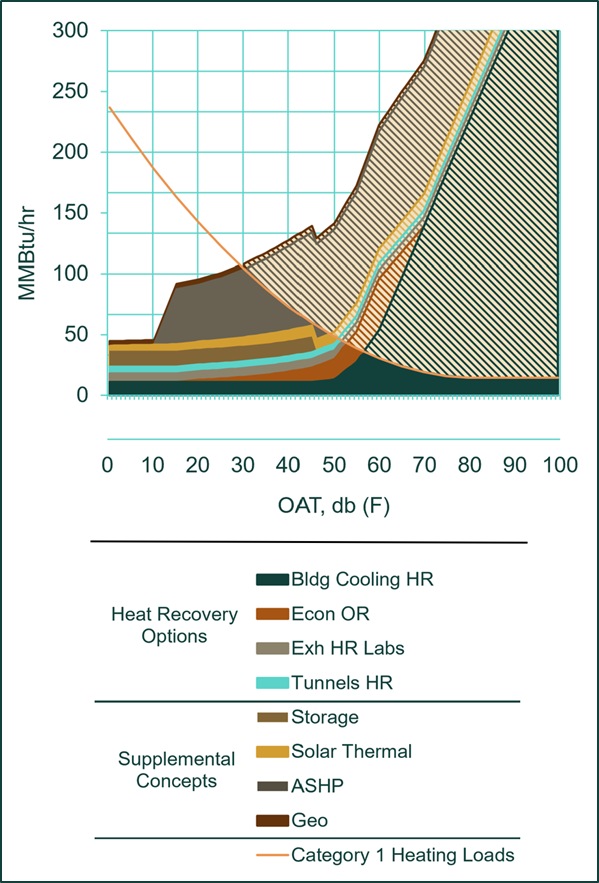
Figure 4 – Integrated Concept for Decarbonizing Category 1 Loads
The heat recovery options are the most cost-effective strategies for decarbonizing the heating load of the campus. If successfully implemented for all applicable buildings and utility tunnels on campus, these four strategies combined have the potential to reduce the total annual steam production by 54%.
The rest of the Category 1 heating loads above 300F outside air dry bulb temperatures represent approximately an additional 25% of the total annual steam production. To decarbonize these loads, four supplemental concepts were outlined in Figure 4. Note that the area beneath the curve in the upper left represents the portion of campus load that will still require steam. This portion represents substantial capacity but a very small portion of annual energy in a typical year (<0.5%). The technologies identified to supplement the demand management of OSU’s CHP alongside the heat recovery chillers are:
- Geoexchange
- Air-Source Heat Pumps (ASHP)
- Solar Thermal
- Thermal Storage
The scale of the supplemental concepts are estimates based on reasonable considerations for future campus planning. The composition of supplemental concept capacities can vary depending on actual technology investment.
Long Term Considerations
The strategies and concepts discussed are all potential options that should be integrated and discussed as part of long-term facility planning on campus. Key initiatives as the campus upgrades buildings should include:
- Ensure buildings are designed or renovated to utilize low-temperature heating water for space heating (less than 1300F).
- Analyze existing buildings that currently do not have cooling or are cooled with water source heat pumps or DX cooling. Analysis will determine if converting to district chilled-water cooling is practical.
- Focus long-term resources on strategies that provide the greatest effect. This includes the heat recovery options in this case study.
- Look for opportunities on new projects where sustainability goals and funding may support supplemental concepts (including ASHP, Storage, Solar Thermal, Geoexchange). Consider establishing goals for implementing some amount of these strategies on each new project.
- Establish policies to provide electric/non-steam equipment and systematic replacement of existing steam and high-temperature end-use equipment (e.g. Category 2 loads).
- Identify mechanical spaces within campus heating/cooling districts that are potential locations for additional centralized chilled- and heating-water production.
- Pursue consistent coordination with local electric utility to ensure they are investing in methods to reduce or abate their own emissions to accommodate local shifts from CHP to electricity in order to globally reduce the campus carbon footprint.
Case Study Contributors: Joe Iaccarino, Dorrie Matthews, Mark Willett, Kory Bowlin
Want to be a part of projects like this? Join Our Team.